Waterborne Transient Electromagnetics
Basic Concept
Transient electromagnetic (TEM) methods, which are alternatively known as time-domain electromagnetics (TDEM), detect variations in subsurface electrical resistivity using electromagnetic (EM) induction. TEM methods are noninvasive and do not require direct electrical contact (i.e., galvanic coupling) with the water or subsurface earth. Thus, TEM methods are ideal for moving or continuous surveys as well as for use at sites with very resistive surface layers that would hinder electrical contact.
Unlike surface TEM methods, waterborne TEM (wTEM) uses equipment that floats on or above the water surface to image the earth materials below a waterbody. wTEM methods induce electric current flow within the subsurface materials, and a returning voltage-decay signal is measured over time after current induction is stopped. wTEM data are filtered to remove noise before being processed using a geophysical inversion to recover layered earth model of apparent resistivity with depth.
Theory
The basic wTEM setup requires at least a single-turn transmitter loop and a multi-turn receiver coil. These coils are typically arranged in offset squares or rectangles, which are shapes chosen for ease of field operation. The transmitter outputs a time-varying direct electrical current (e.g., modified symmetrical square wave) into the transmitter coil. During signal transmission, the current passing through the closed loop of wire creates a primary magnetic field that penetrates the subsurface.
This time-varying magnetic field induces an electromotive force (emf) and subsequent current flow (i.e., eddy current) within subsurface conductors. The induced eddy current generates a secondary magnetic field that opposes the primary field direction and is measured by the receiver coil. Signal transmission is transient, and there periodically exist moments absent of signal. Data are collected during the off times between the bipolar signal transmissions as the electromagnetic energy dissipates into the subsurface.
Current transmission ends abruptly and induces only short-duration emf pulses. This generates a series of smoke ring-like eddy currents that are parallel to and beneath the transmitter loop. The currents penetrate down- and outward while losing amplitude due to material interactions and primary magnetic field dissipation. The decaying current travels through the subsurface and induces voltage pulses in subsurface conductors, which generates more current flow. This cycle continues until the current becomes unmeasurable or decays completely (Nabighian, 1979).
Eddy currents pass slower through conductive materials, generating more current flow and resulting in a slower decay of the secondary magnetic field. Current passes through resistive materials quickly. This causes an increase in current attenuation and a faster decay of the secondary magnetic field. Because the receiver coil is energized by the decaying secondary magnetic field, the measured decay trend can be used to determine the variations of subsurface geoelectrical properties with depth.
Applications
Inversion of individual wTEM measurements produce a one-dimensional (1-D) sounding that displays apparent-electrical resistivity with depth. Multiple soundings can be combined to create two- and three-dimensional (2-D and 3-D) plots of transects or earth volumes, respectively. wTEM depths of investigation (DOI) vary from a few to tens of meters below water surface depending, in part, on geoelectric subsurface properties. Additionally, increasing the magnetic moment (i.e., loop size/current) or the transmission and measurement times increases the DOI.
A variety of equipment is available for stationary one-dimensional wTEM measurements. However, specialized equipment is required to collect continuous or mobile surveys, and few manufacturers sell complete turnkey systems capable of continuous or mobile surveys. TEM is ideal for imaging a conductive body within a resistive medium and, as such, is ideal for recovering earth resistivity in the following waterborne applications:
- Mapping conductive layers or interfaces
- Monitoring Salt-water intrusion
- Identifying potential recharge zones and surface-water/groundwater connection
- Characterizing groundwater resources
- Mapping electrically conductive contaminants
- Identifying submarine groundwater discharge
- Mineral surveying
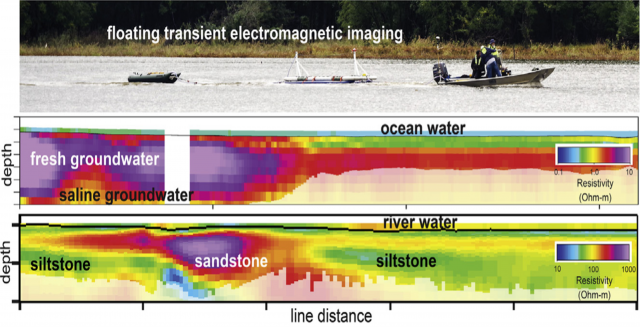
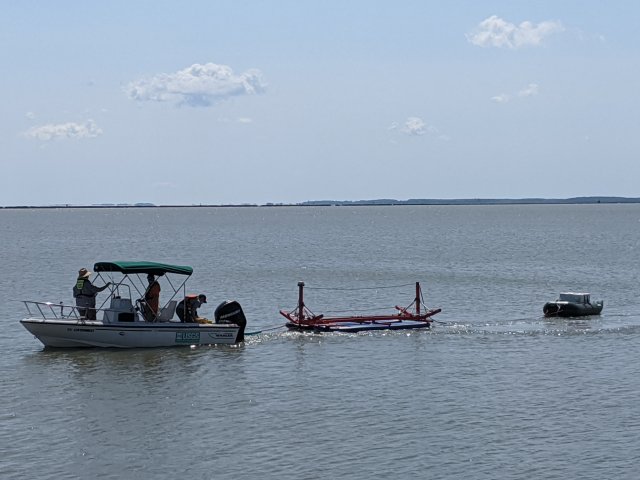
Examples/Case studies
Barrett, B., Heinson,G., Hatch, M., and Telfer, A., 2002, Geophysical Methods in Saline Groundwater Studies: Locating Perched Water Tables and Fresh-Water Lenses: Exploration Geophysics, v. 33, no. 2, p. 115-121, doi:10.1071/EG02115.
Abstract: A fast and informative tool was required for the delineation of stratigraphy and hydrology and for monitoring salinisation effects around the Stockyard Plains Disposal Basin, which is used as a storage lake for salt water pumped from aquifers close to the Murray River near the town of Waikerie, South Australia. Such salinisation effects include the formation of a perched saline watertable close to the disposal basin and consequent threats to local fresh-water lenses. Ground penetrating radar, direct current (DC) resistivity, time-domain EM (TEM) and low induction number frequency-domain EM (FEM) were compared in terms of efficiency and effectiveness at two survey locations near the Stockyard Plains Disposal Basin. Low induction number FEM measurements were fast to implement but suffered from a nonlinear response with ground conductivity in high conductivity areas. Radar methods were ineffective due to high signal attenuation in the highly saline environment. Fast time-sampling TEM successfully depicted a perched saline water table related to leakage from the disposal basin, and was found to be the most useful technique for delineation of hydrogeology due to its high vertical resolution. DC resistivity was the slowest technique, but was found useful in imaging a fresh-water lens. Results from this study suggest that TEM and DC resistivity methods are useful tools for both fresh-water detection and hydrogeology monitoring in saline groundwater environments.
Barrett, B., Hatch, M., Heinson, G., and Tefler, A., 2003, Salinity monitoring of the Murray River using a towed TEM array: ASEG Extended Abstracts, v.2003, no. 2, p. 1-4, doi:10.1071/ASEG2003ab009.
Abstract: Successful implementation of a salt interception schemes (SIS) requires monitoring to determine locations where the scheme needs revision. Current monitoring methods involve near-surface water-salinity measurements, which are affected by water-flow displacement. A survey method that can determine the salinity of water contained in the top few metres of alluvial sediments immediately beneath the river would be a more accurate tool for SIS monitoring. A fast sampling Transient EM technique is investigated as a potential tool for imaging the conductivity of the top 5m of sediment, and thus monitoring the Waikerie SIS in South Australia’s Riverland. A towed TEM array was used to collect 9km of data that shows resistive anomalies correlating with SIS production bores. The system has the advantage of being a small, manageable array and the short inversion time allows same-day interpretation.
Goldman, M., Gvirtzman, H., and Hurwitz, S., 2004, Mapping saline groundwater beneath the Sea of Galilee and its vicinity using time domain electromagnetic (TDEM) geophysical technique: Israel Journal of Earth Sciences, v. 53, no., 3-4, p. 187-197, doi:10.1560/P1W7-UYDE-WJFW-CVHB.
Abstract: An extensive time domain electromagnetic (TDEM) survey covering the Sea of Galilee with a dense grid of points has been recently carried out. A total of 269 offshore and 33 supplementary onshore TDEM soundings were performed along six N-S and ten W-E profiles and at selected points both offshore and onshore along the whole coastal line. The interpreted resistivities were calibrated with the direct salinity measurements in the Haon-2 borehole and relatively deep (5 m) cores taken from the lake bottom. It was found that resistivities below 1 ohm-m are solely indicative of groundwater salinity exceeding 10,000 mg Cl/l. Such low resistivities (high salinities) were detected at depths greater than 15 m below almost the entire bottom of the lake. At some parts of the lake, particularly in the south, the saline water was detected at shallower depths, sometimes at a few meters below the bottom. Relatively high resistivity (fresh groundwater) was found along the margins of the lake down to roughly 100 m, the maximum exploration depth of the system. The detected sharp lateral contrasts at the lake margin between high and low resistivities coincide with the faults separating the carbonate and clastic units, respectively. The geometry of the fresh/saline groundwater interface below the central part of the lake is very similar to the shape of the lake bottom, probably due to the diffusive salt transport from the bottom sediments to the lake water. The above geophysical observations suggest different salt transport mechanisms from the sediments to the central part of the lake (diffusion) and from regional aquifers to the margins of the lake (advection).
Hatch, M., Munday, T., and Heinson, G., 2010, A comparative study of in-river geophysical techniques to define variations in riverbed salt load and aid managing river salinization: Geophysics, v. 75, no. 4, p. 1JA-Z98, doi:10.1190/1.3475706.
Abstract: Increased interest in the character of sediments at the base of waterways, for the purpose of managing river salinization, has led to the application of several geophysical techniques for collecting information from this zone. These instream methods are based on established ground and airborne electrical and electromagnetic technologies, including towed transient electromagnetic systems, towed direct current resistivity array systems, and frequency-domain helicopter electromagnetic systems. Although these systems are individually successful, a systematic examination of their relative effectiveness for identifying variations in substrate conductivity for a common stretch of a river remains lacking. We have compared results obtained from data collected using three instream geophysical techniques for a common stretch of the Murray River in southeastern Australia. The Murray River is an important water resource for drinking and agricultural purposes. Data from these surveys were acquired to locate areas of significant saltwater accession to the Murray from a saline regional groundwater system that discharges into it. The three methods indirectly inform on those reaches that most likely contribute to higher salt loads in the river, and they do this through the identification of a conductive substrate (a gaining reach). For a 25-km stretch of the river, the methods identified similar variations in the conductivity structure of sediment substrate, although differences were observed in the modeled response relating to intrinsic differences between each system, including the sampling interval and resolution. The helicopter electromagnetic (EM) system is capable of acquiring hundreds of kilometers of data in a day, under any river flow condition, from near the river surface to depths in excess of 60m. The other two techniques require safe river flow conditions for acquisition, with as much as 50 river km of data per day possible. The ground-based methods had enhanced lateral and vertical resolving capabilities relative to the helicopter EM system, but their depth of investigation was less (usually only 20m).
Lane Jr., J.W., Briggs, M.A., Maurya, P.K., White, E.A., Pedersen, J.B., Auken, E., Terry, N., Minsley, B., Kress, W., LeBlanc, D.R., Adams, R., and Johnson, C.D., 2020, Characterizing the diverse hydrogeology underlying rivers and estuaries using new floating transient electromagnetic methodology: Science of The Total Environment, v. 740, doi:10.1016/j.scitotenv.2020.140074.
Abstract: The hydrogeology below large surface water features such as rivers and estuaries is universally under-informed at the long reach to basin scales (tens of km+). This challenge inhibits the accurate modeling of fresh/saline groundwater interfaces and groundwater/surface water exchange patterns at management-relevant spatial extents. Here we introduce a towed, floating transient electromagnetic (TEM) system (i.e. FloaTEM) for rapid (up to 15km/h) high resolution electrical mapping of the subsurface below large water bodies to depths often a factor of 10 greater than other towed instruments. The novel FloaTEM system is demonstrated at a range of diverse 4th through 6th-order riverine settings across the United States including 1) the Farmington River, near Hartford, Connecticut; 2) the Upper Delaware River near Barryville, New York; 3) the Tallahatchie River near Shellmound, Mississippi; and, 4) the Eel River estuary, on Cape Cod, near Falmouth, Massachusetts. Airborne frequency domain electromagnetic and land-based towed TEM data are also compared at the Tallahatchie River site, and streambed geologic scenarios are explored with forward modeling. A range of geologic structures and pore water salinity interfaces were identified. Process-based interpretation of the case study data indicated FloaTEM can resolve varied sediment-water interface materials, such as the accumulation of fines at the bottom of a reservoir and permeable sand/gravel riverbed sediments that focus groundwater discharge. Bedrock layers were mapped at several sites, and aquifer confining units were defined at comparable resolution to airborne methods. Terrestrial fresh groundwater discharge with flowpaths extending hundreds of meters from shore was also imaged below the Eel River estuary, improving on previous hydrogeological characterizations of that nutrient rich coastal exchange zone. In summary, the novel FloaTEM system fills a critical gap in our ability to characterize the hydrogeology below surface water features and will support more accurate prediction of groundwater/surface water exchange dynamics and fresh-saline groundwater interfaces.
Mollidor, L., Tezkan, B., Bergers, R., and Löhken, J., 2013, Float-transient electromagnetic method: In-loop transient electromagnetic measurements on Lake Holzmaar, Germany: Geophysical Prospecting, v. 61, no. 5, p. 1056-1064, doi:10.1111/1365-2478.12025.
Abstract: Lake sediments may serve as archives on paleoclimatic fluctuations, geomagnetic field variations and volcanic activities. Lake Holzmaar in Eifel/Germany is a maar lake and its lacustrine sediments provide paleoclimatic proxy data. Therefore, knowledge about the geometry and, especially, about the thickness of the sediments is very important for determining an optimum drilling location for paleoclimatic studies. We have developed a floating in‐loop transient electromagnetic method field set up (Float‐transient electromagnetic method) with a transmitter and receiver size of 18 × 18 m2 and 6 × 6 m2 respectively. This special set up enables in‐loop transient electromagnetic method measurements on the surface of freshwater lakes that define the geometry and the thickness of sediments beneath such lakes thus helping to determine optimum drilling locations. Due to the modular design of the new Float‐transient electromagnetic method field set up, this system can be handled by two operators and can easily be transported. Sixteen in‐loop soundings were carried out on the surface of Lake Holzmaar. The transient electromagnetic method data could not be interpreted by conventional 1D inversions because of the 3D distribution of subsurface conductivity caused by the lake's geometry. Three‐dimensional finite element modelling was applied to explain the observed transients and the 3D conductivity distribution beneath the lake was recovered by taking its geometry into account. The 3D interpretation revealed approximately 55 m thick sediments beneath 20 m deep water in the central part of the lake.
References
Auken, E., Foged, N., Larsen, J.J., Lassen, K.V.T., Maurya, P.K., Dath, S.O., and Eiskjær, T.T., 2018, tTEM — A towed transient electromagnetic system for detailed 3D imaging of the top 70 m of the subsurface: Geophysics, v. 84, no.1, p., 1JF-Z5, doi:10.1190/geo2018-0355.1.
Barrett, B., Heinson,G., Hatch, M., and Telfer, A., 2002, Geophysical Methods in Saline Groundwater Studies: Locating Perched Water Tables and Fresh-Water Lenses: Exploration Geophysics, v. 33, no. 2, p. 115-121, doi:10.1071/EG02115.
Barrett, B., Hatch, M., Heinson, G., and Tefler, A., 2003, Salinity monitoring of the Murray River using a towed TEM array: ASEG Extended Abstracts, v.2003, no. 2, p. 1-4, doi:10.1071/ASEG2003ab009.
Goldman, M., Gvirtzman, H., and Hurwitz, S., 2004, Mapping saline groundwater beneath the Sea of Galilee and its vicinity using time domain electromagnetic (TDEM) geophysical technique: Israel Journal of Earth Sciences, v. 53, no., 3-4, p. 187-197, doi:10.1560/P1W7-UYDE-WJFW-CVHB.
Hatch, M., Munday, T., and Heinson, G., 2010, A comparative study of in-river geophysical techniques to define variations in riverbed salt load and aid managing river salinization: Geophysics, v. 75, no. 4, p. 1JA-Z98, doi:10.1190/1.3475706.
Lane Jr., J.W., Briggs, M.A., Maurya, P.K., White, E.A., Pedersen, J.B., Auken, E., Terry, N., Minsley, B., Kress, W., LeBlanc, D.R., Adams, R., and Johnson, C.D., 2020, Characterizing the diverse hydrogeology underlying rivers and estuaries using new floating transient electromagnetic methodology: Science of The Total Environment, v. 740, doi:10.1016/j.scitotenv.2020.140074.
Maurya, P.K., Christiansen, A.V., Pedersen, J.B., and Auken, E., 2020, High resolution 3D subsurface mapping using a towed transient electromagnetic system - tTEM: case studies: Near Surface Geophysics, v. 18, no. 3, p. 249-259, doi:10.1002/nsg.12094.
Mollidor, L., Tezkan, B., Bergers, R., and Löhken, J., 2013, Float-transient electromagnetic method: In-loop transient electromagnetic measurements on Lake Holzmaar, Germany: Geophysical Prospecting, v. 61, no. 5, p. 1056-1064, doi:10.1111/1365-2478.12025.
Nabighian, M.N., 1979, Quasi-static transient response of a conducting half-space – An approximate representation: Geophysics, v. 44, no. 10, p. 1637-1769, doi:10.1190/1.1440931.